Introduction
Within This Page
This resource page is the first of a series on relevant topics in building science. It is aimed at explaining the key concepts involved in building science, as well as the relationship of this discipline to the architecture / engineering / construction (AEC) industry. It focuses on the systems approach to building technology and the utility of building science to advance the high-performance building agenda.
It should be recognized that prior to the introduction of the systems approach to the discipline of building science, most of research and practice dealt with construction materials and components. The consideration of the entire building system, or in some instances sub-systems, did not emerge until the limits of a less holistic approach became painfully obvious in the form of building defects and failures.
A great deal of research and development toward the advancement of the systems approach remains to be accomplished. Despite their limitations, the system models that have been adopted by modern building science have delivered an overwhelming improvement in the health, safety, and durability of buildings. For this reason, more than any other, building science is now recognized in most developed countries as the core of technical training for architects, explaining why buildings work, why buildings fail, and how to avoid the latter without compromising the artistic, cultural, and environmental qualities of the architectural artifact.
Description
Building Science Defined
Building science is a field of knowledge that draws upon physics, chemistry, engineering, architecture, and the life sciences. Understanding the physical behavior of the building as a system and how this impacts energy efficiency, durability, comfort and indoor air quality is essential to innovating high-performance buildings. Modern building science attempts to work with models of the building as a system, and to apply empirical techniques to the effective solution of design problems.
Neil Hutcheon, the famous Canadian building scientist, defined building science as "a term now widely used, for want of a better one, to describe the growing body of knowledge about the relevant physical science and its application."i
More specifically, contemporary building science is a broad discipline that is concerned with the full life cycle of buildings, including:
- policy (codes and standards);
- planning;
- design;
- construction;
- commissioning;
- facilities management;
- forensics and rehabilitation;
- restoration and retrofit;
- preservation and conservation; and
- demolition (deconstruction) and recycling.
The disciplinary involvement in contemporary building science ranges from the physical and engineering sciences, to economics, political science, behavioral sciences, life sciences, and architecture.ii
The importance of contemporary building science is often fully appreciated after the occurrence of building performance problems, or worse, after failures, rather than at the planning and design stage of building projects. For this reason, contemporary building science has taken on greater importance in response to an increasing trend of innovative departures from traditional building practices based on successful past precedents.
Innovation is not a trial and error process that relies on gradually refining past precedents. It is usually a significant departure from normative practices and relies on the scientific method to advance its agenda. Modern building science, as it is known today, was born of innovation - more correctly, because of the large number of failures encountered when building designers attempted to innovate without applying building science principles. There was no need for building science when only successful precedents were copied and handed down from one generation to the next, but there was also no advancement toward high-performance buildings within traditional building practices.
Building System Theory
Modern systems theory is an expansive body of knowledge with many branches. It is not possible to deal with the full range of theories within these resource pages, but consider these concepts of a system.iii
A system is an integrated assembly of interacting elements, designed to carry out cooperatively a predetermined function. [Gibson 1960]
A system is an integrated network of interacting elements, receiving certain inputs and producing certain outputs, given certain constraints. [Chappelle 1966]
Systems theory, at its fundamental level, is a belief that the world is made up of set(s) of interacting components, and that those sets of interacting components have properties, when viewed as a whole, that do not exist within any of the smaller units. [Allen 1996]
A systems approach is essentially:
- A way of organizing observations;
- A way of thinking about related objects and processes;
- A way of talking about (labeling) the parts (components) of a system; and
- An outcome from systematically considering systemic phenomena.
Systems thinking is an important part of building science because it helps simplify problems by classifying them according to common types. There exist millions of buildings and their diversity would be overwhelming were it not for the systems approach. This approach is derived from general systems theory and the basic characteristics common to all systems are important to keep in mind when applying building science.
Boundaries and Boundary Criteria—Many people believe that a building system ends at the outer surface of its enclosure, in some cases at the property line. But in reality, the building system extends to the outer reaches of what it impacts, and what impacts the building. Basement flooding due to municipal sewer surcharge is an example of the building's plumbing system extending to the local municipal infrastructure services.
Flows and Storage—Inhabitants, energy, water, sewage, and data are examples of the flows and storage characteristics of the building as a system. Occupant behavior is among the most difficult flows to accurately predict in energy models.
Transformations—Buildings age and they are modified by their users, not always in a beneficial way. It is desirable from a life cycle perspective to design buildings that can adapt and adopt new technologies to improve their performance and minimize functional obsolescence.
Spatial and Temporal Hierarchies—Passive survivability and safe/secure building design are premised on achieving spatial and temporal resilience such that the vital functions are maintained both in day-to-day operations and under extreme conditions such as natural and man-made disasters.
Feedback and Control Loops—Buildings are prosthetic extensions of the human body and as such rely on many forms of cybernetics to control the indoor environment and maintain safety and security.
Each of the above characteristics vary in importance, depending on the type of building being designed and its intended use. Building science specialization is often needed to deal with particular aspects of these characteristics (e.g., energy modeling, flood proofing, durability, indoor air quality, blast resistance, etc.). But in all cases, the fundamental understanding of how these characteristics interact may be derived from the building as a system model.
The Building as a System
The idea of the building as a system springs from modern systems theory and the application of building science principles to building behavior and performance.
The building as a system concept is a relatively new development in building science. It resulted directly from the introduction of a systems approach, to building science practice, starting in the 1960s. As innovation increasingly became the means to achieving new forms of architectural expression in the 20th century, analysis and review of building failures indicated that traditional approaches to design were inadequate. This was due to inappropriate adaptations of successful past precedents, or an unknowingly narrow analysis at the building component level for radical departures from technical norms. In both cases the behavior of the whole system was not considered.
The building as a system approach, as depicted in Figure 1, requires designers to explicitly and consciously consider the interactions between the primary elements comprising the system:
- Building enclosure (building envelope system);
- Inhabitants (humans, animals, and/or plants, etc.);
- Building services (electrical/mechanical systems);
- Site, with its landscape and services infrastructure; and
- External environment (weather and micro-climate).
Harmonization of these elements is the key to well-performing buildings.
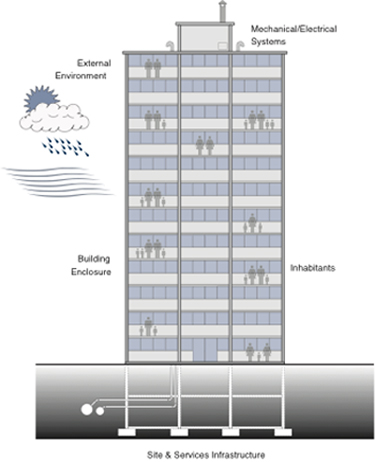
Figure 1. The building as a system model.
Returning to the highly specialized nature of contemporary building science, it is recognized that a large number of materials, components, equipment, and assemblies must be properly integrated to achieve a high-performance building. At the same time, it must be appreciated that most performance problems involve the building enclosure, which also represents the primary passive environmental control system. In view of these considerations, this Resource Page is largely focused on the building science underlying building enclosures and how they are influenced by climate and weather. A comprehensive listing of design parameters may be found in the Resource Page on Building Enclosure Design Principles and Strategies.
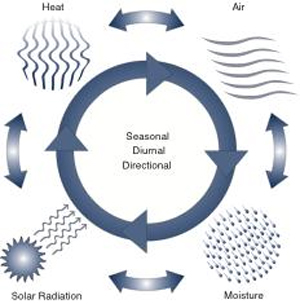
Figure 2. Physical mechanisms driving the behavior of the building as a system.
Recognizing that the physical forces affecting structural integrity must always be adequately resolved, there remain four primary physical mechanisms associated with climate and weather that drive the behavior of the building as a system in terms of its role as a moderator of the indoor environment (see Figure 2):
Heat Flow - the conductive, convective, and radiative flow of heat;
Air Flow - the air flow across and within the building enclosure due to air leakage and ventilation;
Moisture Flow - the flow of water and vapor across and within the building enclosure; and
Solar Radiation - the influence of insulation on the opaque and transparent enclosure components.
In the building as a system, all of these physical mechanisms are occurring in various combinations at various times. During cold periods, heat and warm moist air escape through leaks in the building enclosure. To compensate, the heating system must supply the amount of heat being lost, and to replace the lost moisture, the indoor air must be humidified for occupant health and comfort. During hot periods, heat and warm moist air are driven into the building and the HVAC system must cool and dehumidify. Under all conditions, the building enclosure must manage the heat, air, and moisture flows. The occupants can exert as great an influence as the climate through their activities. This explains why a building may be very fit for one occupancy (e.g., warehouse or factory), but then experience problems when the occupancy changes (e.g., residential or institutional). Problems occur when the balance of moisture, heat, and air flows is disturbed beyond the performance thresholds of the building as a system.
The key to the fitness of a building is the balanced control of these physical mechanisms, so that durability, comfort, energy efficiency, indoor air quality, health, and safety are not compromised.
Building Performance
The term "performance" may be defined as the level of service provided by a building material, component, or system, in relation to an intended, or expected, threshold or quality.
For example, the structural performance of a building may be judged in terms of its resistance to dead, live, soil, wind, hydrostatic, and seismic loads as prescribed by applicable codes. Within the established thresholds for these loads, the structure would be required to behave adequately according to expectations in terms of strength, durability, deflections, and vibrations.
When the intended or expected level of performance is not achieved, the resultant behavior is termed a "failure" which must not be confused with the term "defect", a minor damage or blemish which has no immediate or significant impact on performance, and which may be suitably repaired.
An important contribution of building science is the quantification of performance parameters such that many of these can be predicted at the design stage, and assessed / confirmed after the building is occupied and operational. This preoccupation with prediction and validation has led to the appreciation of the need for a systems approach, as building scientists grapple with issues such as indoor air quality and sustainable buildings.
The significant thing to remember about inadequate building performance is that it results in the vast majority of litigation, and the application of building science via the systems approach is among the more effective preventive measures against failures and defects. It is also a highly useful diagnostic tool when assessing the condition of existing buildings that are candidates for restoration and retrofit. In order to deploy the systems approach in the design and assessment of buildings, it is first necessary to establish a framework of performance requirements. The section which follows deals with the ongoing development of such a framework based on the pioneering work of the late Neil Hutcheon, former Director of the Division of Building Research at the National Research Council Canada.iv Figure 3 depicts a hierarchy of performance requirements derived from building science principles.v
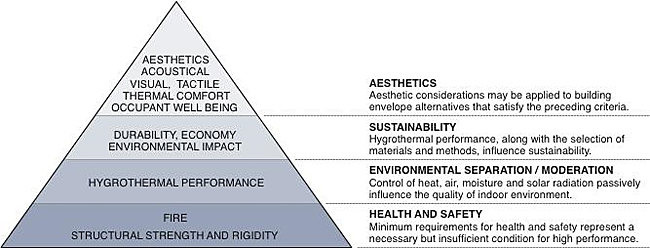
Figure 3. Building science hierarchy of performance requirements.
To borrow from mathematical terminology, building science is a necessary but insufficient condition for great architecture. However, its pivotal supporting role cannot be underestimated in importance. The vast majority of litigation between parties to construction projects involves the inadequate performance of building elements that are intended to provide firmness and commodity. Inferior delight is still only punishable by the rebukes of peers and critics, and has so far eluded the grasp of litigation and legislation.
The concept of a building performance framework is intended to explicitly represent:
- External and internal conditions affecting a building system (e.g., climate, weather, site, soils, occupancy, and indoor climate class);
- Parts and inter-relationships comprising a building system (e.g., the behavior of materials, components, equipment and sub-systems);
- Parameters or indicators defining acceptable performance (e.g., aesthetics, health and safety, economy, sustainability, etc.); and
- Methods, tools, and techniques for designing and analyzing performance according to the parameters, inter-relationships and conditions cited above.
Context for Contemporary Building Performance Objectives
Appropriate building performance objectives involve numerous interfaces between the building, its occupants, and the natural and built environment as depicted in Figure 4. Some of these interfaces cannot be regulated (e.g., climate, weather, human physiology, etc.), while others may be manipulated within some prescribed range (e.g., energy efficiency, affordability, etc.). The terms of reference for the regulated aspects of buildings remain in a constant state of flux, as evidenced by the many changes associated with each development cycle of building codes and standards. And the context for building performance in relation to the environment, the economy, and occupant expectations has also changed appreciably in developed countries.
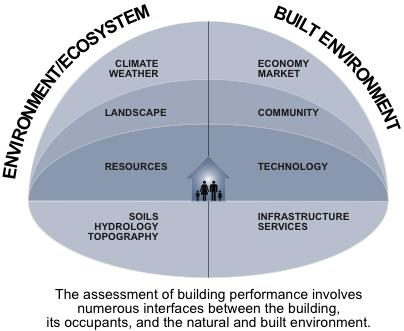
Figure 4. Contemporary context for building performance objectives.
The most significant change in recent times has been the awareness of the ecological impacts of buildings. Today, society recognizes that the building as a system does not arbitrarily end at the property line, and may have far reaching environmental implications. It is reasonable to expect there will continue to be a variety of interpretations of the relative importance (priority) of the factors involved in the performance of building systems.
Rather than dwell on the global scale of building performance concepts and parameters, this discussion will focus on physical aspects of performance that may be objectively quantified and compared.
Relationship between Physics, Materials, Components, and Systems
Performance concepts in building codes and standards have existed largely as constraints guiding the prescriptive codes and standards development process. One of the major challenges in developing an effective building performance objectives framework has been the establishment of explicit parameters supported by building science knowledge, and specialized knowledge from allied disciplines. These are premised on the relationship between physical phenomena and building system behavior.
A building is a system which consists of materials, components (assemblies, equipment), sub-systems, and systems that interact with physical phenomena in the process of providing an intended level of performance to its immediate occupants and societal stakeholders. Focusing on physical phenomena from a building science perspective, the relationship of the constituent elements of a building system and these physical phenomena is depicted in Figure 5.
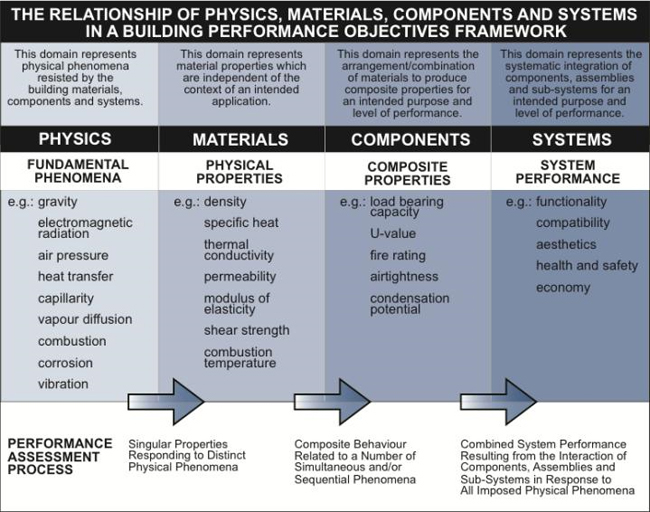
Figure 5. Physics, materials, components, and systems.
The key points to appreciate from this relationship are as follows:
The fundamental physical phenomena imposed on a material, component, or system drive its response (behavior).
The suitability of a material, component, or system must, as a minimum, adequately address the imposed physical phenomena.
The complexity of problems increases dramatically as the design process proceeds from selecting materials, to arranging components, to integrating systems.
The relationship can also be used in reverse, figuratively speaking, to assess the suitability of an existing building system to another set of physical conditions which differ from those originally considered in its design.
Due to the multi-functional nature of components and sub-systems (e.g., a wall may provide structural support, fire safety, and moderation of the environment), it is important to relate constituent elements of the building to a coherent hierarchy of objectives. The hierarchy of physics, materials, components, and systems is a practical means of dealing with performance objectives at the conceptual level, recognizing that it may bear little, if any, resemblance to the actual intellectual process (design). The importance of differentiating between representations of relationships, and the actual reasoning processes which make use of these representations, cannot be underestimated. Research in the fields of artificial intelligence and expert systems has demonstrated that the linkages between knowledge representation and its application require sophisticated interpretation.vi Presumably, the cultivation of sophisticated interpretive skills remains one of the more critical roles for the higher education of design professionals.
A Conceptual Model of Building Behavior
Building behavior (performance) is a highly complex, resultant phenomenon. It involves numerous simultaneous and sequential physical phenomena, and the response of the building as a system will vary depending on the nature and arrangement of the constituent elements. At present, a comprehensive, explicit model of building system behavior has not been developed, but is assumed to exist in some implicit form among the collective of building industry experts. A helpful reminder of the challenge associated with developing a comprehensive system model was provided by Hutcheon (Hutcheon and Handegord, 1983, pp.3-4):
"The design of buildings has been, and still is, to a large extent, based on building practice. Changes have been slow and, in the main, have come about through an evolutionary process of trial and error. Building practice has been fundamentally an inheritance from the past, modified by factors such as climate, economy, social habits, local aesthetic values, and local resources of materials and skills. The evolutionary process works slowly under the influence of new factors; it is equally slow in rejecting the obsolete.
The growth of scientific knowledge has led to great advances in the analysis and rational design of the purely structural functions of a building. There has also been a great deal of development in individual materials and components. As yet, there have been relatively small advances in dealing adequately with all of the combinations of elements and with the complex interrelationships of phenomena involved in the performance of an entire building. The reasons are not hard to find. It is sufficient to note that, even now, contemporary building science draws on the knowledge and experience of almost every branch of engineering science.
We have long since passed the point where we are content to rely on the "trial-by-use" method of assessing changes in design, materials and construction. Many new and interesting materials, systems and methods of design and construction are offered each year. Those responsible for assessing and screening such new developments realize only too well the relative inadequacy of our present knowledge of the suitability of any given material or method. In addition, our standards of performance are continually being raised. As we reduce our major difficulties in turn, minor ones assume greater relative proportions, and we clamour for their reduction or elimination also, in the name of progress. The increasing state of knowledge appears less and less adequate as the demands upon it increase."
Since the time of Hutcheon's observation, a stronger need for a whole system model of building performance has been recognized within the building science discipline. While a broadly accepted model continues to elude building science researchers and practitioners, some advances have been made in various aspects of performance, such as wetting/drying potential of enclosures, window performance, etc. At the conceptual level, approaches such as the general limit states design model have been applied to structural design, however, this approach is not well suited to many areas of building performance (e.g., access and egress, room dimensions, etc.) and the gathering of data may not always be possible even where the model is applicable (e.g., statistics for water leakage in basements).
Given this situation, an attempt to construct a general, extensible schema of building behavior applicable to the whole system, as well as its constituent elements, is presented in Figure 6. At this point, a comprehensive application of the schema to whole building system performance remains to be completed.
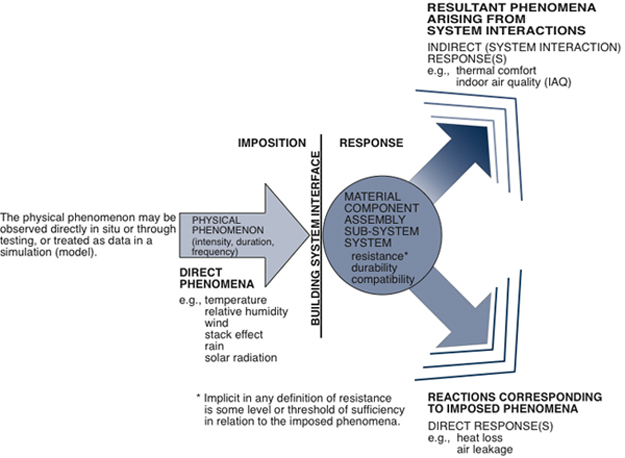
Figure 6. Schema describing physical building behavior.
Based on the schema depicted in Figure 6, it may be noted that by linking a number of simple schemata, complex behavior may be described, if not quantitatively modeled. In the example phenomena depicted in Figure 6, the direct response of the building enclosure to the temperature, air pressure, and humidity difference between the indoor and outdoor environments results in heat loss and air leakage. The indirect response influences thermal comfort and indoor air quality if the enclosure provides low effective thermal resistance (excessive thermal bridging and/or insufficient insulation) and the condensation of moisture promotes mold growth. This approach is useful in constructing more sophisticated models of whole system behavior to aid designers with fundamental performance considerations and system interactions.
At this point in the development of contemporary building science, computational methods for the design and assessment of building systems have not emerged. It has been argued that the integrative process of design will remain an exclusively human task, and that conceptual models in the form of computational technologies will only serve as learning aids and practice guidelines. Regardless of the future of building science, a great deal of progress has been made toward developing simple models of building behavior and arranging them within a framework of performance objectives. The next section presents one such approach to the comprehensive assessment of the physical performance of building systems
A Building Performance Objectives Framework
The framework depicted in Figure 7 is an extension of earlier work pursued by the author.vii It is based on the premise that in the assessment or design process, the key consideration appears to be the performance objective or intent. This view has since been strongly reinforced as many nations move towards objective-based building codes.viii
An interesting aspect of any objective-based framework is that the intent remains constant while the means of achieving the intent or objective continue to evolve with advances in technology. It appears humans will always expect buildings to provide firmness, commodity, and delight, and that architects will always have to find appropriate means of responding to their clients' demands.
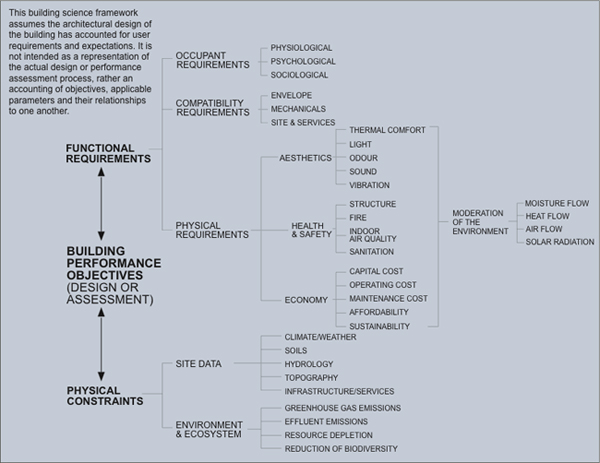
Figure 7. Building performance objectives framework from a building science perspective.
The framework presented in Figure 7 consists of two primary elements:
- The physical constraints which are imposed by site conditions and the limits or thresholds of the global environment and local ecosystem; and
- The functional requirements of buildings that encompass occupant requirements, compatibility requirements, and physical requirements.
Contemporary building science supports the societal objective of sustainable architecture by balancing the physical constraints and the functional requirements, ideally without compromising architectural aesthetics and high performance.
The predominant area of interest for building science is under functional requirements, and within this area further and more specific objectives are identified that constitute the basis for designing and/or assessing physical building system performance.
This, or any performance objectives framework, is intended to provide designers with an explicit means of accounting for compliance with the functional requirements of buildings. While it is possible that future developments in computer technology may automate many aspects of design and assessment,ix it is doubtful that designers will assign significant responsibility to prosthetic applications for assuring due diligence, simply because software cannot shoulder liability.
Finally, if nothing else, this framework exposes the breadth of building science and reinforces the realization that its mastery will require a lengthy commitment to study and practice.
Building System Integration
A common purpose of building science is to achieve building system integration, not by-trial-and-error over many generations of building precedents, but each and every time a building is being designed and built. This implies defining a level of performance and a means of assuring compliance.
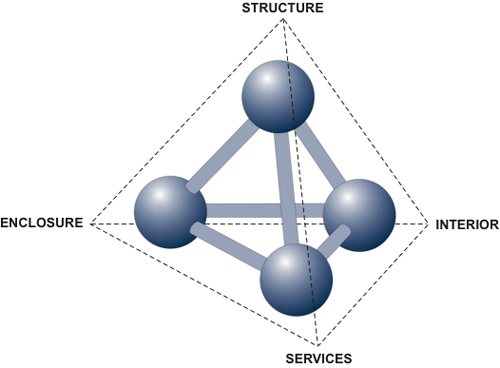
Figure 8. Building system integration involves the building structure, its enclosure (envelope), the interior elements, and the building services (i.e., mechanical, electrical, etc.).
Optimizing performance goes beyond compatibility between the structure, enclosure, interior, and services. It involves the assessment of economic, social, and environmental parameters so that performance targets are attained affordably within the skill capacity of the industry. This effectively means innovation may be defined as achieving better performance and higher quality at less cost over the life cycle of a building or facility.
Applied building science research has indicated the control of moisture in building enclosure design generally takes precedence over other control measures simply because so many of the requirements for the control of heat transfer, air leakage and solar radiation are satisfied when all forms of moisture have been carefully considered. At the time of developing this Resource Page, energy efficiency is a primary goal of most developed nations, and this objective is not compromised by designing building enclosures to manage moisture. The levels of thermal insulation needed to avoid interstitial condensation leading to durability problems are equal to, or higher, than those required to provide cost effective levels of energy efficiency over the life cycle of a building. This is the rationale behind a subsequent set of building science Resource Pages beginning with moisture management in building enclosures.
A number of related Resource Pages are also dedicated to explaining how various performance objectives identified herein may be achieved, but for the purposes of understanding building science concepts, it is important to appreciate that the building enclosure, or envelope, is the primary environmental separator/moderator. It performs a passive role, unlike mechanical and electrical systems, that actively supplement the amount of heat, air, moisture, and daylight the enclosure is unable to provide. When all active systems fail, the building enclosure is the last line of defense between the indoors and the outdoors. High-performance building enclosures provide passive sustainability during extreme weather phenomena and natural disasters, and safely shelter their inhabitants.
Synopsis
In summary of the ideas and relationships that have been presented, the following conclusions may be considered:
Buildings are systems that must be appropriately integrated by designers to achieve defined levels of performance.
Building science provides a disciplined means of dealing with the physical requirements of buildings that is completely compatible with the architectural design and building construction processes.
Innovation in modern architecture relies on building science and the systems approach to ensure that building performance meets the expectations of building owners, inhabitants, and society.
The context for building performance has more recently evolved to include issues of ecology and sustainable development. This expansion of performance parameters, coupled with increasing consumer expectations, has dramatically increased the complexity of buildings. Performance objectives frameworks and conceptual models have become necessary methodologies to assure all aspects of the integration of well performing building systems have been carefully addressed.
For further study on this topic read the concluding chapter to Robert Mark's compendium on Architectural Technology Up to the Scientific Revolution wherein he observes:
"By focusing on a wide range of historic buildings, from their below-ground-level foundations to the peak of their timber roofs, the typical developmental pattern of pre-scientific technology becomes evident. Much of what was then learned through experience and observation is available to today's designers from scientific analysis."x
Contemporary building science has evolved beyond simple analysis and now offers a range of sophisticated design tools, testing protocols, and performance simulation/validation techniques. These have become invaluable supplements to professional experience and the critical observation of actual building performance. The role of contemporary building science within architecture and engineering continues to reinforce the dynamic relationship between theory and practice in the AEC industry.
Additional Resources
Publications
- Building Science for a Cold Climate by Neil Hutcheon and Gus Handegord. Institute for Research in Construction, 1983.
- The Building Systems Integration Handbook, edited by Richard D. Rush. The American Institute of Architects, 1986.
- Building Science for Building Enclosures by John Straube and Eric Burnett. Building Science Press, December 2005.
- Integrated Buildings: The Systems Basis of Architecture by Leonard R. Bachman. John Wiley and Sons, 2003.
Online Sources of Building Science Information
Building science information can be found among a number of sources, such as conference proceedings, journals, studies, and various technical publications made available through government, industry and academia. The sources cited herein represent organizations or institutions that are predominantly involved with building science. For example, sources such as the American Society of Civil Engineers will have a part of their publications dedicated to building science, but for the most part, focus on traditional civil engineering topics. These kinds of organizations have not been listed, but may contain highly relevant building science information.
- Building Science Centre of Excellence Research Database
- Building Science Corporation
- Canada Mortgage and Housing Corporation—Accessible and Adaptable Homes
- Lawrence Berkeley Laboratory—Building Energy Efficiency
- National Institute of Building Sciences
- National Institute of Standards and Technology—Buildings and Construction
- National Renewable Energy Laboratory—Buildings Research
- Natural Resources Canada—Energy Efficiency for Buildings
- Oak Ridge National Laboratory—Building Technologies Program
In addition to the sources cited above, keyword searches on the Internet will yield a number of references for a particular topic. Product manufacturer organizations and government departments dedicated to buildings, or aspects of buildings such as energy efficiency, are also potential sources of invaluable building science information. In order to obtain maximum benefit from these myriad sources of building science information, it is important to gain a foundation of fundamental building science concepts and terminology.
References
i. Building Science for a Cold Climate, NRCC 39017 by Hutcheon, N. B. and Gus Handegord. Ottawa: 1983.
ii. Fields of Research in Building Science (brochure) by Education Liaison Committee. Washington, DC: Building Research Institute, 1963.
iii. 3 Definitions as found in: Hierarchy theory: a vision, vocabulary, and epistemology by Timothy F. H. Allen with Valerie Ahl, illustrated by Paula Lerner. New York: Columbia University Press, 1996.
iv. Fundamental Considerations in the Design of Exterior Walls for Buildings, Technical Report No. 13, Division of Building Research by Hutcheon, N. B. Ottawa: National Research Council Canada, 1953.
v. Development of a Wall Performance Classification System by Kesik, T. and David De Rose. Toronto ON: CIB World Building Congress 2004, May 2–7, 2004.
vi. Knowledge in the Form of Patterns and Neural Network Computing Knowledge Engineering, Volume I, Fundamentals by Pao, Y.H., H. Adeli (editor). New York: McGraw Hill, 1990.
vii. A Knowledge Based Systems Approach to the Assessment of Building Performance Proceedings of the Second Canadian Conference on Computing in Civil Engineering, pp. 469-480 by Kesik, T. and K.A. Selby. Ottawa, Ontario: 1992.
viii. Objective Based Codes: A New Approach for Canada by Canadian Commission on Building and Fire Codes. Ottawa: February 1996.
ix. Towards Integration of Service Life and Asset Management Tools for Building Envelope Systems Proceedings of the Seventh Conference on Building Science and Technology, pp. 153-163 by Lacasse, M.A., D.J. Vanier, B.R. Kyle. Toronto: 1997.
x. Architectural Technology Up to the Scientific Revolution by Mark, Robert (editor). Cambridge, Massachusetts: MIT Press, 1993.