Introduction
Within This Page
Existing buildings may often require retrofits for explosive threats based on changes in mission, occupancy, or threat level. Conducting a threat, vulnerability, and risk assessment is the first step in determining the need to upgrade a conventionally designed building in order to protect its occupants and assets. This will identify the maximum credible threats and the associated hazards based on the site conditions, building layout, access control, structural framing, and facade components.
The effectiveness of the upgrades depends to a great extent on the structural details of the building and the aesthetic and functional impacts that may be tolerated. For example, historic preservation requirements may limit secure design alternatives that would otherwise be considered appropriate. The cost of protective design and the impact of this protection on the property may be minimized by using advanced analytical methods. These methods, developed over years of explosive testing and numerical simulation, enable the design team to focus resources on portions of the structure that are most likely to sustain damage and minimize materials required to mitigate these hazards. The protective measures that may benefit most from these methods involve the design of protective facade systems, the hardening of structures to resist the effects of progressive collapse, and the retrofit of existing structures. Therefore, the following is a description of retrofit upgrades that may be used to either reduce the hazard of debris impact or to strengthen the structure to resist loads that the original design did not consider.
Description
A. Anti-Shatter Film
Anti-shatter film (ASF), also commonly known as "shatter-resistant window film" (SRWF) or "security film," is a laminate used to improve post-failure performance of existing windows. Applied to the interior face of glass, anti-shatter film holds the fragments of broken glass together in one sheet, thus reducing the projectile hazard of flying glass fragments. A more appropriate name for anti-shatter film would be "fragment reduction" film, since the methodology behind this hazard mitigation technique is focused upon retaining glass fragments resulting from blast overpressures.
Most anti-shatter films are made from polyester-based materials and coated with adhesives. Anti-shatter films are available as clear and tinted. Clear ASFs have minimal effects on the optical characteristics of the glass; tinted ASFs can increase the effectiveness of existing heating/cooling systems (see also WBDG Windows and Glazing and High-Performance HVAC) while providing a variety of aesthetic and optical enhancements. Most ASFs are designed with solar inhibitors to screen out ultraviolet (UV) rays, although over time the UV absorption damages the film and degrades its effectiveness. Abrasions on the face of tempered glass can reduce the glass strength, but ASFs are also available treated with an abrasion-resistant coating that can prolong the life of tempered glass.
ASFs are packaged on rolls that are generally available in widths as small as 24 inches and as large as 72 inches, depending on the manufacturer. Some manufacturers laminate multiple layers of film together in order to enhance performance. Whether one-ply or multi-ply, the overall film thickness can range from 2 to 15 mils. According to some government criteria (and verified by published test results), a 7–mil thick anti-shatter security film or specially manufactured 4–mil thick film, is considered to be the minimum thickness required to provide effective response to blast loads.
There are three types of anti-shatter film installation methods. They are:
- Daylighting Installation
- Wet Glazed Installation
- Mechanical Anchored Installation
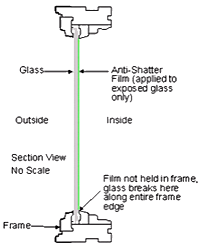
Typical daylight installation of anti-shatter film
Daylighting Installation: The application of security film must, at a minimum, cover the clear area (a.k.a. the portion of the glass unobstructed by the frame) of the window. This minimum application to the exposed glass without any means of attachment or capture within the frame, termed "daylight" installation, is commonly used for retrofitting windows. Application of the film to the edge of the glass panel where it would cover the glass within the bite is called an edge to edge installation, and is often used in conjunction with dry glazing installations. Other methods of application may improve film performance and further reduce hazards but are typically more expensive to install, especially in retrofits.
Energy-absorbing catch systems, used in conjunction with a daylight application of anti-shatter film, are another mechanism for retaining and reducing debris hazards. Cables spanning across the window will impede the flight of filmed glass and absorb a considerable amount of energy upon impact. These cable catch systems have demonstrated through explosive testing to be more efficient and effective than the more rigid catch bar systems described in Section D below.
Wet Glazed Installation: The wet glazed installation is a system where the film is positively attached to the frame using a high strength liquid sealant such as silicone. Frequently used for field retrofits, the method allows the flexible frame to deform slightly, reducing glass fragments entering the building and offering more protection than the daylight installation. The wet glazed installation system is more costly than the daylight installation system, but is less expensive than the mechanically attached or anchored installation system described below.
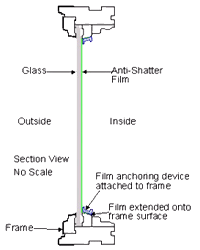
Typical mechanically anchored installation of anti-shatter film
Mechanically Anchored/Attached Installation: Anti-shatter film is most effective when it is used in conjunction with a blast-tested anchorage system. While a film may be effective in keeping glass fragments together, it may not be particularly effective in retaining the glass in the frame. Securing the film to the frame with a mechanically connected anchorage system further reduces the likelihood of the glazing system exiting the frame. Mechanical anchorage systems employ screws and/or batten strips to attach the film to the frame along 2 or 4 sides. Because additional framework is necessary, the mechanical attachment method can be less aesthetically pleasing than the wet glazed installation system.
All application and attachment methods can be installed on-site in either steel or aluminum frames. While some mechanically attached systems may be used for a wide variety of windows, others are designed for a particular type of window frame. Certain types of window frames may require a custom fabricated anchorage system.
In addition to considering the various methods of installation, the designer must consider the thickness of the film and the task of positioning the film on the glass. A lighter weight or thinner film eases installation. Water used to aid in positioning the film during application must be thoroughly extruded as the film is not very permeable and moisture that does not dry will prevent the development of the full adhesive bond strength. Anti-shatter film should be carefully examined and chosen for its physical, optical, and thermal characteristics, with special consideration given to the adhesive used, the window thickness, and the window area. Also, window frame systems must be capable of transferring the load collected by the glazing system. Corner welded frames are preferred over frames constructed of individual components.
The analytical tools that evaluate the likely performance of façades in response to blast loads are used to demonstrate compliance with established blast criteria or performance specifications. Many of these performance specifications contain the criterion that the façade must be a balanced design. The objective of this criterion is to realize the capacity of all the materials, maximize the potential energy dissipated due to deformation, and manage the failure mechanisms. This is accomplished by assuring a controlled sequence of failure. The performance criteria of the existing façade systems will determine the effectiveness of the existing mullions, connections between the different components, and the anchorages to the structure.
For more information on anti-shatter films, see WBDG Glazing Hazard Mitigation and Designing Buildings to Resist Explosive Threats.
B. Laminated Glass
Laminated glass consists of two or more pieces of glass permanently bonded together by a tough plastic interlayer made of polyvinyl butyral (PVB) resin. Once sealed together, the glass "sandwich" behaves as a single unit. Annealed, heat strengthened, tempered glass, or polycarbonate glazing can be mixed and matched between layers of laminated glass in order to design the most effective light for a given application. When fractured, fragments of laminated glass tend to adhere to the PVB interlayer rather than fall freely and potentially cause injury.
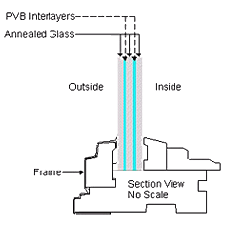
Section view of a typical laminated window. "UK testing experience on laminated glass has shown that at ranges of perhaps 50m or more, buildings subject to vehicle bombs could be given façades (including windows) which would keep the blast pulse or the major part of it, out without rupture. Such windows would be with a designed thickness of laminated glass securely retained in purpose-designed substantial frames, and the fa¸ade framing would be designed to survive the deflections incurred, albeit with substantial residual deformations" DC Smith, 1997
Laminated glass usually lasts as long as ordinary glass if it is not broken or damaged in any way. The correct installation of laminated glass is very important to ensure long life. Manufacturers typically recommend installation of laminated glass on 1/4" setting blocks that are at least 6 inches long and placed at the quarter points of the pane. Architectural details should incorporate a weep system for exterior glazing systems. Regardless of the degree of protection required from the window, laminated glass needs to be installed with proper sealant to necessitate that no water comes in contact with the edges of the glass. The sealant supplier should verify that the sealant and PVB interlayer are compatible. Generic sealants shown to be compatible with PVB are the polysulfides, silicones, butyl, or polybutene tapes, and polyurethanes. Minimum face and edge clearance should be provided as required by the manufacturer. It should be noted that field cutting should be kept to a minimum in butt glazing installations to minimize edge defects. Glazing guidelines, such as those presented in the Glass Association of North America Glazing Manual should be followed to avoid installation problems. Typical allowances for glass, metal, and erection tolerance, expansion and contraction should be made.
Energy-absorbing catch systems may also be used in conjunction with laminated glass to impede the flight of the glass and absorb a considerable amount of energy upon impact. As mentioned, these cable catch systems have been demonstrated through explosive testing to be more efficient and effective than the more rigid catch bar systems described in Section D below.
Laminated glass requires the same maintenance as ordinary glass. That is, the glass should be cleaned with a soft, grit-free cloth soaked in a mild soap, detergent, or slightly acidic cleaning solution and rinsed immediately with water. Excess rinse water should be promptly removed with a clean squeegee. Excessive application of strong solvents should be avoided.
For more information on laminated glass, see WBDG Glazing Hazard Mitigation and Designing Buildings to Resist Explosive Threats.
C. Blast Curtains
Blast curtains are attached to the interior frame of a window opening and essentially catch the glass fragments produced by a blast wave. The debris is then deposited on the floor at the base of the window. The use of these curtains does not eliminate the possibility of glass fragments penetrating the interior of the occupied space, but instead limits the travel distance of the airborne debris. Overall, the hazard level to occupants is significantly reduced by the installation of blast curtains. However, a person sitting directly adjacent to a window outfitted with a blast curtain may still be injured by shards of glass in the event of an explosion.
Blast curtains are made from a variety of materials, including a warp knit fabric or a polyethylene fiber. The fiber can be woven into a panel as thin as 0.029 inches that weighs less than 1.5 ounces per square foot. This fact dispels the myth that blast curtains are heavy sheets of lead that completely obstruct a window opening and eliminate all natural light from the interior of a protected building.
The main components of any blast curtain system are the curtains themselves, the attachment mechanism by which the curtain is attached to the window frame, and either a trough or another retaining mechanism at the base of the window to hold the excess curtain material. Blast curtains, with curtain rod attachment and sill trough, differ largely from one manufacturer to the next. The curtain fabric, material properties, method of attachment, and manner in which they operate also vary, providing many options within the overall classification of blast curtains. These facts make blast curtains applicable in many situations.
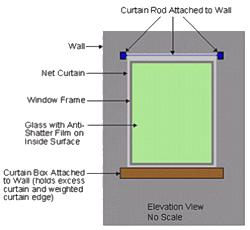
Elevation view of a typical blast curtain
Blast curtains differ from standard curtains in that they do not open and close in the typical manner. Blast curtains are designed to remain in a closed position at all times. The curtain may be pulled away from the window to allow for cleaning, blind or shade operation, or occupant egress in the case of fire. However, the curtains can be rendered ineffective if the building occupants do not use them correctly by pulling them away from the glazing. The color and openness factor of the fabric contributes to the amount of light that is transmitted through the curtains and the see-through visibility of the curtains. While the color and weave of these curtains may be varied to suit the aesthetics of the interior décor, the appearance of the windows is altered by the presence of the curtains.
The curtains may either be anchored at the top and bottom of the window frame or anchored at the top only and outfitted with a weighted hem. The curtain needs to be extra long with the surplus either wound around a dynamic tension retainer or stored in reservoir housing. When an explosion occurs, the curtain feeds out of the receptacle to absorb the force of the flying glass fragments. The effectiveness of the blast curtains relies on their use.
D. Glazing Catch Cable/Bar Retrofit
Rigid Catch Bar Systems
As explained earlier, laminated glazing is designed to hold the glass shards together as the window is damaged. Yet, unless the window frames and attachments are upgraded to withstand the capacity of the laminated glass, there is a high possibility that entire sheets of glass would fly free of the window frames in a blast environment. Rigid catch bar systems have been designed and tested as a means of increasing the effectiveness of laminated window upgrades. The rigid catch bars intercept the laminated glass and disrupt their flight.
Rigid catch systems collect huge forces upon impact and require substantial anchorage into very substantial structure to prevent failure. If either the attachments or the supporting structure are incapable of restraining the forces, the catch system will be dislodged and become part of the debris. Alternatively, the debris may be sliced by the rigid impact, severely limiting the effectiveness of the catch bars.
Flexible Catch Bar Systems
Flexible catch bars can be designed to absorb a significant amount of the energy upon impact, thereby keeping the debris intact and impeding their flight into occupied spaces. They also may be designed to repel the debris from the failed glazing as well as the walls in which the windows are mounted. The debris restraint system must be strong enough to withstand the momentum transferred upon impact; and the connections must be capable of transferring the forces to the supporting slabs and spandrel beams. Under no circumstances should the design of the restraint system add significant amounts of mass to the structure because it could be dislodged and present an even greater risk to the occupants of the building.
Energy-Absorbing Catch Cable Systems
The use of cable systems has long been recognized as an effective means of stopping massive objects moving at high velocity. Cables are extensively used to absorb significant amounts of energy upon impact and their flexibility makes them easily adaptable to many situations. The diameter of the cable, the spacing of the strands, and the means of attachment are all critical in designing an effective catch system. These catch cable concepts have been used by protective design window manufacturers as restraints for laminated lights. To confirm the adequacy of the cable catch system to restrain the debris resulting from an explosive event, an analytical simulation or a physical test is required.
High-Performance, Energy-Absorbing Cable Catcher Systems retain glass and frame fragments and limit the force transmitted to the supporting structure. These commercially available retrofit products consist of a series of 1/4" diameter stainless steel cables connected with a shock-absorbing device to an aluminum box section, which is attached to the jambs, the underside of the header, and topside of the sill. The energy-absorbing characteristics allow the catch systems to be attached to relatively weakly constructed walls without the need for additional costly structural reinforcement. To reduce the possibility of slicing the laminated glass, the cable may either be sheathed in a tube or an aluminum strip may be affixed to the glass directly behind the cable.
For more information on energy-absorbing catch cable systems, see WBDG Glazing Hazard Mitigation and Designing Buildings to Resist Explosive Threats.
E. Polymer Materials for Structural Retrofit
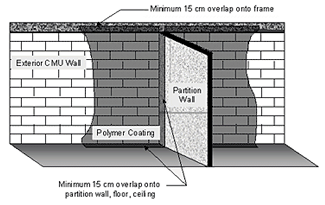
Polymer coating
Unreinforced masonry walls provide limited protection against air blast due to explosions. When subjected to overload from air blast, brittle unreinforced CMU walls will fail and the debris will be propelled into the interior of the structure, possibly causing severe injury or death to the occupants. This wall type has been prohibited for new construction where protection against explosive threats is required. Existing unreinforced CMU walls may be retrofitted with a sprayed-on polymer coating to improve their air blast resistance. This innovative retrofit technique takes advantage of the toughness and resiliency of modern polymer materials to effectively deform and dissipate the blast energy while containing the shattered wall fragments. Although the sprayed walls may shatter in a blast event, the elastomer material remains intact and contains the debris.
The blast mitigation retrofit for unreinforced CMU walls consists of an interior (and an optional exterior) layer of polyurea applied to exterior walls and ceilings. The polyurea provides a ductile and resilient membrane that catches and retains secondary fragments from the existing concrete block as it breaks apart in response to an air blast wave. The effectiveness of a spray-on polymer coating has been demonstrated through explosive testing at the Air Force Research Laboratory and by extensive numerical laboratory simulation.
For more information on the use of polymer materials for resisting explosive threats, see WBDG Designing Buildings to Resist Explosive Threats.
F. Geotextile Fabric Retrofit
In lieu of the elastomer, an aramid (Geotextile) debris-catching system may be attached to the structure by means of plates through-bolted through the floor and ceiling slabs. Similar to the elastomer retrofit, the aramid layer does not strengthen the wall, but instead it restrains the debris that would otherwise be hurled into the occupied spaces.
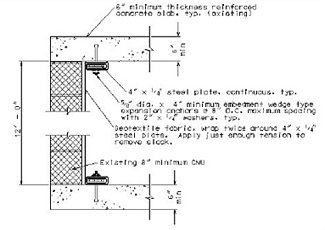
Geotextile fabric retrofit cross section
G. Structural Retrofit
In some cases, it may not be possible to retrofit an existing building to limit the extent of collapse to one floor on either side of a failed column. If the members are retrofitted to develop catenary—the natural curve created by a flexible cord freely suspended between two fixed points—behavior, the adjoining bays must be upgraded to resist the large lateral forces associated with this mode of response. This may require more extensive retrofit than is either feasible or desirable. In such a situation, it may be desirable to isolate the collapsed region rather than risk propagating the collapse to adjoining bays.
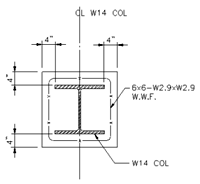
Typical steel column concrete encasement
The retrofit of existing structures to protect against a potential progressive collapse resulting from the detonation of a terrorist explosive threat may therefore best be achieved through the localized hardening of vulnerable columns. These columns need only be upgraded to a level of resistance that balances the capacities of all adjacent structural elements. At greater blast intensities, the resulting damage would be extensive and termed global collapse rather than progressive collapse. Attempts to upgrade the structure to conform to the alternate path method will be invasive and potentially counterproductive. Care must be taken not to weaken a structure in the attempt to make it more robust.
Conventionally designed columns may be vulnerable to the effects of explosives, particularly when placed in contact with their surface. Standoff elements such as partitions and enclosures may be designed to guarantee a minimum standoff distance; however, this alone may not be sufficient. A steel jacket or a carbon fiber wrap may be used to provide additional resistance to reinforced concrete structures. These systems effectively confine the concrete core, increase the confined strength and shear capacity of the column, and hold the rubble together to permit it to continue carrying the axial loads. The capacity of steel flanged columns may be increased with a reinforced concrete encasement that adds mass to the steel section and protects the relatively thin flange sections. The details for these retrofits must be deigned to resist the specific weight of the explosives and the standoff distance. See also WBDG Designing Buildings to Resist Explosive Threats, section on Column Reinforcements.
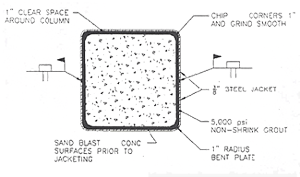
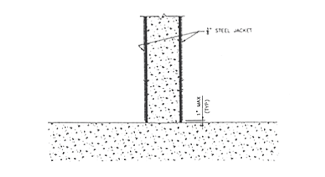
Typical concrete jacketed column
Section of typical steel jacketed concrete column
Floor slabs are typically designed to resist downward gravity loading and have limited capacity to resist uplift pressures or the upward deformations experienced during a load reversal. Therefore, floor slabs that may be subjected to significant uplift pressures, which may overcome the gravity loads and subject the slabs to reversals in curvature, require tension reinforcement at the top fiber of the mid-span locations and bottom tension reinforcement at the underside near the supports. If the slab does not contain this tension reinforcement, it must be supplemented with a lightweight carbon fiber application that may be bonded to the surface at critical locations. Carbon fiber reinforcing mats bonded to the top surface of slabs would strengthen the floors for upward loading and reduce the likelihood of slab collapse from blast infill uplift pressures as well as internal explosions in mailrooms or other susceptible spaces. This lightweight high tensile strength material will supplement the limited capacity of the concrete to resist these unnatural loading conditions. These retrofit options are currently the subject of a Technical Support Working Group (TSWG) research project, being performed by Weidlinger Associates (WAI) at the Energetic Materials Research and Test Center (EMRTC), and the initial results show the retrofits to be effective. An alternative approach is to notch grooves into the top of the concrete slabs, and then to epoxy carbon fiber rods into those grooves. Although this approach may offer greater capacity, it is much more invasive and has not been evaluated with explosive testing. See also WBDG Designing Buildings to Resist Explosive Threats, section on Floor Slab Reinforcements.
Relevant Codes and Standards
Federal standards and criteria are widely recognized as the primary source of guidelines for upgrading and retrofitting of existing buildings to resist explosive threats. Because of the uniqueness of each building's existing conditions and physical security design requirements, there are limited codes and standards that apply to retrofitting for blast mitigation.
Federal Guidelines
- Department of Defense
- FM 3-19.30 Physical Security—Sets forth guidance for all personnel responsible for physical security
- Unified Facility Criteria (UFC) 1-200-01, Design: General Building Requirements
- Unified Facilities Criteria (UFC) 4-010-01, DoD Minimum Anti-Terrorism Standards for Buildings—Establishes prescriptive procedures for threat, vulnerability, and risk assessments and security design criteria for DoD facilities.
- Department of Homeland Security
- General Services Administration (GSA)
- PBS-P100 Facilities Standards for the Public Buildings Service - Chapter 8, Other "official use only" documents may be obtained from the Office of the Chief Architect.
- Department of State
- Architectural Engineering Design Guideline (5 Volumes) (limited official use only)
- Physical Security Standards Handbook, 07 January 1998. (limited official use only)
- Structural Engineering Guidelines for New Embassy Office Buildings, August 1995. (limited official use only)
Private Sector Guidelines
- Blast Effects on Buildings: Design of Buildings to Optimize Resistance to Blast Loading by G.C. Mays and P.D. Smith. London: Thomas Telford Publications, 1995.
- ACI 318 Building Code Requirements for Structural Concrete and Commentary, Chapter 21, American Concrete Institute
Public Testing Institutions
Private Testing Laboratories
Many private laboratories with expertise in protective glazing systems testing are also available. Contact the Protective Glazing Council for additional information and referral.
Additional Resources
Security Criteria Centers
- Defense Threat Reduction Agency—Department of Defense (DOD) Anti-terrorism body—Pentagon's J34
- Department of Homeland Security Federal Protective Service
- Naval Facilities Engineering Command (NAVFAC), Engineering and Expeditionary Warfare Center
- U.S. Army Corps of Engineers, Protective Design Center
- U.S. Department of Defense
Organizations
- American Concrete Institute (ACI)
- American Institute of Steel Construction (AISC)
- American Society of Civil Engineers, (ASCE)—for papers and guideline related to blast design in a structural engineering publications database
- Federal Facilities Council (FFC) Standing Committee on Physical Security & Hazard Mitigation
- Federal Emergency Management Agency (FEMA)—offering guidance on man-made disaster threat assessment and mitigation planning
- Glass Association of North America (GANA)
- International Window Film Association (IWFA)
- Protective Glazing Council
- Safety Glazing Certification Council (SGCC)
- The Infrastructure Security Partnership (TISP)
Publications
- Anti-Terrorism: Criteria, Tools & Technology by Joseph L. Smith, Applied Research Associates
- Architectural Design for Security and Security and Technology Design by Donald M. Rochon. June 1998.
- Designing for Crime and Terrorism, Security and Technology Design by Randall I. Atlas. June 1998.
- Safety/Security Window Film by the International Window Film Association
- Security Glazing Specification by The Protective Glazing Council